
Kissimmee Homeschooling

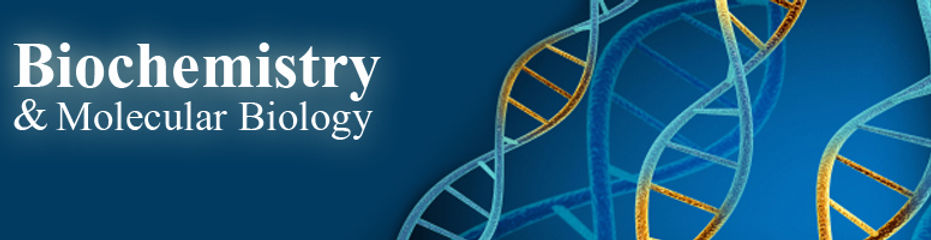
Biochemistry & Molecular Biology
Learning Biochemistry & Molecular Biology
What is Biochemistry?
Biochemistry, sometimes called biological chemistry, is the study of chemical processes within and relating to living organisms. By controlling information flow through biochemical signaling and the flow of chemical energy through metabolism, biochemical processes give rise to the complexity of life.
Over the last decades of the 20th century, biochemistry has become so successful at explaining living processes that now almost all areas of the life sciences from botany to medicine to genetics are engaged in biochemical research.[2] Today, the main focus of pure biochemistry is in understanding how biological molecules give rise to the processes that occur within living cells, which in turn relates greatly to the study and understanding of whole organisms.
Biochemistry is closely related to molecular biology, the study of the molecular mechanisms by which genetic information encoded in DNA is able to result in the processes of life. Depending on the exact definition of the terms used, molecular biology can be thought of as a branch of biochemistry, or biochemistry as a tool with which to investigate and study molecular biology. Much of biochemistry deals with the structures, functions and interactions of biological macromolecules, such as proteins, nucleic acids, carbohydrates and lipids, which provide the structure of cells and perform many of the functions associated with life. The chemistry of the cell also depends on the reactions of smaller molecules and ions. These can be inorganic, for example water andmetal ions, or organic, for example the amino acids which are used to synthesize proteins. The mechanisms by which cells harness energy from their environment via chemical reactions are known as metabolism. The findings of biochemistry are applied primarily in medicine, nutrition, and agriculture. In medicine, biochemists investigate the causes and cures of disease. In nutrition, they study how to maintain health and study the effects of nutritional deficiencies. In agriculture, biochemists investigate soil and fertilizers, and try to discover ways to improve crop cultivation, crop storage and pest control.
Biochemistry and the Machinery of Life
Biochemistry is one of the crossover fields of chemistry. Biochemists have to understand both the living world and the chemical world. Even if you don’t want to become a biochemist, you'll still have to understand atomsand molecules as a biologist.
The key thing to remember is that biochemistry is the chemistry of the living world. Plants, animals, and single-celled organisms all use the same basic chemical compounds to live their lives. Biochemistry is not about the cells or the organisms. It's about the smallest parts of those organisms, the molecules. It's also about the cycles that create those biological compounds.
Intro to Biochemistry
Repeating Biochemical Cycles
You can probably guess that biochemical cycles repeat over and over. Those cycles allow living creatures to survive on Earth. It could be the constant process of photosynthesis that creates sugars in plants or building complex proteins in the cells of your body. Also, cycles rely onenzymes and other proteins to move the atoms and molecules. Understanding the helper molecules is as important as learning about the cycles themselves.
Every cycle has a place, and each one is just a small piece that helps an organism survive. In each cycle, molecules are used as reactants and then transformed into products. Life is one big network of activity where each piece relies on all of the others. A compound, such as an herbicide, may only break one part of one cycle in a plant. However, because everything needs to work together, the whole plant eventually dies.
Start with the Basics
We may have been talking about cycles to this point. However, we think it's important that you understand the different types of molecules you will find in biochemistry. We aren't going to go into the citric acid cycle and its ten steps. We won't even look at the eleven steps involved in the breakdown ofglucose. At your level of understanding, it's enough to know the difference between a steroid, an amino acid, and a carbohydrate. There will be plenty of time for you to memorize the pathways and the movement of molecules during each step of a cycle.
Biochemistry and Organic Chemistry
Some of you may have heard about organic chemistry. It's a much broader field than biochemistry. It looks at every molecule that might have a carbon atom. You could be studying plastics, cellulose, gasoline, fats in your cells, or paint. Organic chemistry looks at many carbon-containing compounds beyond the ones found in living systems.
We like biochemistry because we learn about things that are inside of us. We can relate to what happens when we eat and how our bodies are constructed. We can imagine how the molecules are moving around the mitochondria or chloroplasts, as opposed to chemical changes that make natural gas. If you choose a career in biology or chemistry, you will need to understand the information in both biochemistry and organic chemistry. Why? Because the movement of atoms in the bio-chem world follows the same rules you will learn in o-chem.
Metabolism
Metabolism is such a big word to explain a simple idea. We all need energy to survive. Whether we are plants, animals, or bacteria, we all need energy. Energy doesn't just float around in a form we can use to survive. We need to eat (mainly sugars) and digest food. That process of chemical digestion and its related reactions is called metabolism. Metabolism is the total of all the chemical reactions an organism needs to survive.
Sounds a lot like biology. Why is it here in biochemistry? There are two main chemical processes that make our world go round, involving two simple chemical reactions. The first is called glycolysis. That's the breakdown of sugars. The second process is called photosynthesis. That is the series of reactions that builds sugars. You need to remember that the overall metabolism of an organism includes thousands of chemical reactions. The reactions in glycolysis and photosynthesis are just the cornerstones to life.
Building Up
First, you need to build up the molecules that store energy. We'll start with photosynthesis. It's no use explaining the breakdown of sugars without telling you how they were made:
LIGHT (Energy) + CO2 + H2O --> C6H12O6 + O2
You will only find this reaction in plants and algae (maybe some bacteria). They take sunlight and combine carbon dioxide (CO2) and water (H2O). Then they create glucose (C6H12O6) and oxygen gas (O2). Chemists say that they are fixing the atmospheric carbon (C). Remember, plants put the energy in glucose. Glucose is in most of the foods you eat, and the oxygenyou breathe comes from those plants. Even if you have a piece of meat, that animal was originally able to get its glucose from a plant. You need to understand just how important plants are to you and the rest of life on Earth.
Breaking Down
Respiration is a three-step process that includes glycolysis, the Krebs cycle, and a bunch of electrons being pushed around the membranes of mitochondria. Together they take that energy out of the sugar-related molecules. Glucose is combined with oxygen and releases usable energy, carbon dioxide, and water.
C6H12O6 + O2--> Usable Energy (ATP) + CO2 + H2O
Cells can use that extra energy to power their functions. The energy isn't just floating around. It's stored in an excitable compound called ATP (adenosine triphosphate). ATP is the power molecule used by all the cells of an organism to power the secondary reactions that keep us alive. You may also hear about other power molecules like NADH, NADPH, or FADH. These are equally important as ATP, but they are used less often. We exhale carbon dioxide when we breathe. That CO2 comes from the breakdown of glucose in our mitochondria. As we just told you, plants can take in that carbon dioxide and use it to make sugars. Did you know that plants also create CO2? They might not breathe it out the way we do, but plants need energy too. They break down sugars in their cells and release CO2 just like us.
Cycles Around You
There are many types of cycles inside living organisms and across the Earth. One of these is called the carbon cycle. It's a large cycle that involves the movement of carbon (C) through living organisms and the non-living environment. Plants are a good starting point when looking at the carbon cycleon Earth. Plants have a process calledphotosynthesis that enables them to take carbon dioxide (CO2) out of the atmosphere and combine it with water (H2O). Using the energy of the Sun, plants make sugars (C6H12O6) and oxygen (O2) molecules. It's a process called carbon fixation. The carbon dioxide gas is fixed into more stable and usable organic molecules. All of the non-photosynthetic creatures on the planet use the oxygen and sugars created by plants. Even plants use the oxygen to survive when they break down their own sugars for energy.
Another part of the carbon cycle happens when organisms (plant and animal) break sugars down into energy, water, and carbon dioxide. Animals are the non-photosynthetic creatures of the planet. They are not able to create their own food. Instead, they eat plants or other animals. The process of metabolism breaks down the sugars, proteins, and starches they eat. The results are energy for the organism, water, and carbon dioxide molecules. The carbon dioxide then returns to the atmosphere where the plants can use it again.
So, your carbon dioxide is now back in the atmosphere. Cars and all internal combustion engines also create carbon dioxide when they burn gasoline. All of that CO2 gas goes into the atmosphere. While some of it is taken up by plants on the land and microorganisms in the oceans, it seems to be building up over the past few decades. Is it just because of humans, or is it natural? While there are natural changes in carbon dioxide levels, there is no doubt that all of our machines are adding to the buildup. We are also destroying many photosynthetic organisms as we harvest trees for wood or pollute the oceans of the Earth. As far as you are concerned, don't worry about your breathing. You can huff and puff all you want and it won't change the amount of CO2 in the atmosphere. If humans are putting extra CO2 into the air, it's from our machines and unmanaged environmental actions.
Small Cycles
There are also smaller cycles that happen in individual cells. The Krebs cycle is a label used to describe the citric acid cycle that occurs in the mitochondria. This smaller cycle takes a molecule called pyruvate (CH3-CO-COO-) and pulls energy out of it. Carbon dioxide molecules are released during the process. We told you about plants that fix carbon dioxide in the overall carbon cycle. Those carbon compounds are changed one piece at a time in the Calvin cycle in the chloroplasts of plants. You will discover smaller cycles in the synthesis, or creation, of amino acids. Humans can make many of their own amino acids, which will be used to build proteins. Sometimes we don't have a cycle to make a specific amino acid. To get that essential amino acid into our body, we have to find a plant or animal and eat it. That's just the way life works.
Sweet, Sweet Carbs
Carbohydrate is a fancy way of saying "sugar." Scientists came up with the name because the molecule have manycarbon (C) atoms bonded to hydroxide (OH-) groups. Carbohydrates can be very small or very large molecules, but they are still considered sugars. Plants can create long chains of these molecules for food storage or structural reasons.
What's It Used For?
A carbohydrate is called an organic compound, because it is made up of a long chain of carbon atoms. Sugars provide living things with energy and act as substances used for structure. When sugars are broken down in themitochondria, they can power cell machinery to create the energy-rich compound called ATP (adenosine triphosphate). Some examples of structural uses might be the shell of a crab (chitin) or the stem of a plant (cellulose). We'll talk about them in a little bit.
Saccharides
Scientists also use the word saccharide to describe sugars. If there is only one sugar molecule, it is called a monosaccharide. If there are two, it is a disaccharide. If there are three, it is a trisaccharide. You get the idea.
Simple Sugars
What about the simplest of sugars? A sugar called glucose is the most important monosaccharide on Earth. Glucose (C6H12O6) is created byphotosynthesis and used in cellular respiration. When you think of table sugar, like the kind in candy, it is actually a disaccharide. The sugar on your dinner table is made of glucose and another monosaccharide called fructose(C6H12O6). These sugars have the same numbers of atoms, but they are different structures called isomers.
Polysaccharides
When several carbohydrates combine, it is called a polysaccharide ("poly" means many). Hundreds of sugars can be combined in a branched chain. These chains are also known as starches. You can find starches in foods such as pasta and potatoes. They are very good sources of energy for your body.
Sugars for Structure and Support
An important structural polysaccharide iscellulose. Cellulose is found in plants. It is one of those carbohydrates used to support or protect an organism. Cellulose is in wood and the cell walls of plants. You know that shirt you're wearing? If it is made of cotton, that's cellulose, too! There can be thousands of glucose subunits in one large molecule of cellulose. If we were like some herbivores or insects, such as termites, we could eat cellulose for food. Those animals don't actually digest the polysaccharides. They have small microorganisms in their bellies that break down the molecules and release smaller sugars.
Polysaccharides are also used in the shells (chitin) of crustaceans, such as crabs and lobsters. Chitin is similar in some ways to the structure of cellulose, but has a far different use. The shells are solid, protective structures that need to be molted (left behind) when the crustacean begins to grow. It is very inflexible. On the other hand, it is very resistant to damage. While a plant may burn, it takes very high temperatures to hurt the shell of a crab. If you know the way crabs are cooked, you know that the crab meat cooks on the inside of the shells when it is boiled. There is no damage to the shells at the temperature of boiling water (H2O at 100oC).
Many Forms of Lipids
Lipids are another type of organic molecule. Remember that organic means they contain carbon (C) atoms. It's not like organic farming at all. When you think of fats, you should know that they are lipids. Lipids are also used to make steroids and waxes. So, if you pick out some earwax and smell it, that's a lipid, too!
Get the Wax Out of Your Ears
Waxes are used to coat and protect things in nature. Bees make wax. It can be used for structures, such as the bees' honeycombs. Your ears make wax. It is used to protect the inside of your ear. Plants use wax to stopevaporation of water from their leaves. There is a compound called cutinthat you can find in the plant cuticle covering the surface of leaves. It helps to seal and protect plant structures. Don't worry about plants being able to breathe. There are still small holes that let gases in and out of the leaves.
Steroids
Steroids are found in animals within something called hormones. The basis of a steroid molecule is a four-ring structure: one ring with five carbons and three rings with six carbons. You may have heard of steroids in the news. Many bodybuilders and athletes have used anabolic steroids to build muscle mass. The steroids make their bodies add more muscle than they would normally be able to. Those anabolic steroids help bodybuilders wind up stronger and bulkier (but not faster). Steroids are also used in necessary medicines. Some help people with acne, while others are used as muscle relaxers for injuries.
NOTE: Never take drugs to enhance your body. Those athletes are actually hurting their bodies. They can't see it, because it is slowly destroying their internal organs and not the muscles. When they get older, they can have kidney and liver problems. Some even die.
Triglycerides
Fat is also known as a triglyceride. It is made up of a molecule known asglycerol that is connected to one, two, or three fatty acids. Glycerol is the basis of all fats and is made up of a three-carbon chain that connects the fatty acids together. A fatty acid is just a long chain of carbon atoms connected to each other.
Saturated and Unsaturated
There are two kinds of fats, saturated and unsaturated. Unsaturated fats have at least one double bond in one of the fatty acids. A double bond happens when four electrons are shared or exchanged in a bond. They are much stronger than single bonds with only two electrons. Saturated fats have no double bonds.
Fats have a lot of energy stored up in their molecular bonds. That's why the human body stores fat as an energy source. When you have extra sugars in your system, your body converts them into fats. When it needs extra fuel, your body breaks down the fat and uses the energy. Where one molecule of sugar only gives a small amount of energy, a fat molecule gives off many times more.
The Nucleic Acids
The nucleic acids are the building blocks of living organisms. You may have heard of DNA described the same way. Guess what? DNA is just one type ofnucleic acid. Some other types are RNA, mRNA, and tRNA. All of these "NAs" work together to help cells replicate and build proteins. NA? Hold on. Might that stand for nucleic acid? It might.
While you probably don't have to remember the full words right now, we should tell you that DNA stands fordeoxyribonucleic acid. RNA stands forribonucleic acid. The mRNA and tRNA are messenger RNA and transfer RNA, respectively. You may even hear about rRNA which stands for ribosomal RNA. They are called nucleic acids because scientists first found them in the nucleus of cells. Now that we have better equipment, nucleic acids have been found in mitochondria, chloroplasts, and cells that have no nucleus, such as bacteria and viruses.
The Basics
We already told you about the biggie nucleic acids (DNA, mRNA, tRNA). They are actually made up of chains of base pairs of nucleic acids stretching from as few as three to millions. When those pairs combine in super long chains (DNA), they make a shape called a double helix. The double helix shape is like a twisty ladder. The base pairs are the rungs. We're very close to talking about the biology of cells here. While it doesn't change your knowledge of the chemistry involved, know that DNA holds your genetic information. Everything you are in your body is encoded in the DNA found in your cells. Scientists still debate how much of your personality is even controlled by DNA. Back to the chemistry...
Five Easy Pieces
There are five easy parts of nucleic acids. All nucleic acids are made up of the same building blocks (monomers). Chemists call the monomers "nucleotides." The five pieces are uracil, cytosine, thymine,adenine, and guanine. No matter what science class you are in, you will always hear about ATCG when looking at DNA. Uracil is only found in RNA. Just as there are twenty (20) amino acids needed by humans to survive, we also require five (5) nucleotides.
These nucleotides are made of three parts:
1. A five-carbon sugar
2. A base that has nitrogen (N) atoms
3. An ion of phosphoric acid known as phosphate (PO43-)
DNA Details
DNA stands for deoxyribonucleic acid. It is special, because it holds the code for every cell in your body. That's right. Every cell in your body uses DNA as an instruction manual. If you want to take away the importance of that statement, you can say that DNA is just a long spiral chain ofnucleotides. But it's more. So much more.
So, you get all of those nucleotides in two long chains that twist around each other. That twisting shape is called a double helix. The spiral ladder has the ability to wind and unwind so that the nucleic acidchain can duplicate itself. That duplication process, called replication, happens every time a cell divides.
DNA Down Time
When a cell is in its normal state, the DNA is not duplicating and it just looks like a blob of white strands. Scientists use the word chromatin to describe that DNA. Chromatin can be found in several levels of organization. It changes its overall structure (physically, not chemically) during different times of the cell's life. The nucleic acid chains usually sit around uncoiled as loose strands. When it is time for the cell to reproduce, they condense and wrap up very tightly. The tightly wound DNA is called a chromosome. Chromosomes look kind of like long, limp hot dogs. They are also found in pairs.
In most organisms, you will find DNA in the nucleus. Chromosomes work with other nucleic acids in the cell to build proteins and help in cell replication. You will most likely find mRNA (messenger-ribonucleic acid) in the nucleus with the DNA. tRNA (transfer ribonucleic acid) is found outside of the nucleus, floating in the cell. In a few organisms calledprokaryotes, there is no defined nucleus and the DNA is found throughout the cell.
Your DNA Instruction Manual
Just remember that DNA is the instruction manual for an organism. Even though all organisms use the same five nucleic acid to build and replicate DNA, it is the order that makes us who we are. You've got the threepyrimidine bases — cytosine, thymine, and uracil — and the two purine bases — adenine and guanine. Every organism on Earth has a different number and order of base pairs. A base pair is created when one purine base bonds to a pyrimidine base. The order of base pairs determines your physiology. When you get a group of three base pairs of DNA, they can code for one amino acid. Once the string of mRNA leaves the nucleus with the information, long chains of amino acids can be constructed. That's when you have made proteins that can do work for the cell. It all goes back to the number and order of base pairs in the original DNA strand.
Acids in Proteins?
The first thing you might be asking is, "What is an amino acid?" There are more than fifty, and each one of them is a little different. Amino acids are used in every cell of your body to build the proteins you need to survive. All organisms need some proteins, whether they are used in muscles or as simple structures in the cell membrane. Even though all organisms have differences, they still have one thing in common: the need for basic chemical building blocks.
Amino acids have a two-carbon bond. One of the carbons is part of a group called the carboxyl group (COO-). A carboxyl group is made up of one carbon (C) and two oxygen (O) atoms. That carboxyl group has a negative charge, since it is a carboxylic acid (-COOH) that has lost itshydrogen (H) atom. What is left — the carboxyl group — is called a conjugate base. The second carbon is connected to the amino group. Amino means there is an NH2 group bonded to the carbonatom. In the image, you see a "+" and a "-". Those positive and negative signs are there because, in amino acids, one hydrogen atom moves to the other end of the molecule. An extra "H" gives you a positive charge.
Making Chains
Even though scientists have discovered over 50 amino acids, only 20 are used to make something called proteins in your body. Of those twenty, nine are defined as essential. The other eleven can be synthesized by an adult body. Thousands of combinations of those twenty are used to make all of the proteins in your body. Amino acids bond together to make long chains. Those long chains of amino acids are also called proteins.
Essential Amino Acids: Histidine, Isoleucine, Leucine, Lysine, Methionine, Phenylalanine, Threonine, Tryptophan, and Valine.
Nonessential Amino Acids: Alanine, Asparagine, Aspartic Acid, Glutamic Acid.
Conditional Amino Acids: Arginine (essential in children, not in adults), Cysteine, Glutamine, Glycine, Proline, Serine, and Tyrosine.
Something Called Side Groups
The side groups are what make each amino acid different from the others. Of the 20 side groups used to make proteins, there are two main groups: polar and non-polar. These names refer to the way the side groups, sometimes called "R" groups, interact with the environment. Polar amino acids like to adjust themselves in a certain direction. Non-polar amino acids don't really care what's going on around them. The polar and nonpolar chemical traits allow amino acids to point towards water (hydrophilic) or away from water (hydrophobic). The growing chains can then begin to twist and turn when they are being synthesized.
Building Proteins from Amino Acid Chains
We already showed you some information about amino acids. Proteins are made of amino acids. Even though a protein can be very complex, it is basically a long chain of amino acid subunits all twisted around like a knot.
Primary Structure
As proteins are being built, they begin as a straight chain of amino acids. This chain structure is called the primary structure. Sometimes chains canbond to each other with two sulfur (S) atoms. Those bonds would be called a disulfide bridge.
Secondary Structure
After the primary structure comes the secondary structure. The original chain begins to twist. It's as if you take a piece of string and twist one end. It slowly begins to curl up. In the amino acid chain, each of the amino acids interacts with the others and it twists like a corkscrew (alpha helix) or it takes the shape of a folded sheet (beta sheet). We talked about amino acids that are hydrophobic and hydrophilic. Those desires to stay away or be close to water (H2O) play a part in the twisting.
Tertiary Makes Step Three
Let's move on to the tertiary structure of proteins. By now you're probably getting the idea that proteins do a lot of folding and twisting. The third step in the creation of a protein is the tertiary structur. The amino acid chains begin to fold even more and bond using more bridges (the disulfide bridges).
Quaternary Is Fourth and Final
We can finally cover the quaternary structure of proteins. Quaternary means four. This is the fourth phase in the creation of a protein. In the quaternary structure, several amino acid chains fromthe tertiary structures fold together in a blob. You heard us right. "Blob" is the term we use on this site. They wind, entwined, in and out of each other. Some of the most famous protein blobs are hemoglobin in human red blood cells and the photosystems in plant chloroplasts.
Enzymes Make the World Go 'Round
We have a whole section where we tell you about reactions and the molecules that change in those reactions. Chemical bonds are being created and destroyed over a series of many intermediate reactions. Those changes rarely happen on their own when you look at biological systems.
Will a blob of protein in a Petri dish simply break down into amino acids? No. To break a protein down into its amino acids you will need enzymes. Enzymes are biological molecules (proteins) that act as catalysts and help complex reactions occur everywhere in life. Let’s say you ate a piece of meat. Proteases would go to work and help break down the peptide bonds between the amino acids.
Will all enzymes break down all substances? No. Enzymes are very specific catalysts and usually work to complete one task. An enzyme that helps digest proteins will not be useful to break down carbohydrates. Also, you will not find all enzymes everywhere in the body. That would be inefficient. There are unique enzymes in neural cells, intestinal cells, and your saliva.
Assembly Line Robots
You all know about cars and the assembly lines where they are made. There are giant robots helping people do specific tasks. Some lift the whole car, some lift doors, and some put bolts on the frames. Enzymes are like those giant robots. They grab one or two pieces, do something to them, and then release them. Once their job is done, they move to the next piece and do the same thing again. They are little protein robots inside your cells.
The robot that was designed to move a car door can't put brakes on the car. The specialized robot arms just can't do the job. Enzymes are the same. They can only work with specific molecules and only do specific tasks. Because they are so specific, their structure is very important. If only one amino acid of the enzyme is messed up, the enzyme might not work. It would be as if someone unplugged one of the cords in a robot.
For example, some herbicides are used to block plant enzyme activity. A tiny herbicide molecule can attach to the active site of an enzyme and stop it from working. Plants have adapted by changing one or two amino acids in their enzymes. They adjust their structure, are able to continue working, and the herbicide can no longer limit the enzyme.
Four Steps of Enzyme Action
1. The enzyme and the substrate are in the same area. Some situations have more than one substrate molecule that the enzyme will change.
2. The enzyme grabs on to the substrate at a special area called the active site. The combination is called the enzyme/substrate complex. Enzymes are very, very specific and don't just grab on to any molecule. The active site is a specially shaped area of the enzyme that fits around the substrate. The active site is like the grasping claw of the robot on the assembly line. It can only pick up one or two parts.
3. A process called catalysis happens. Catalysis is when the substrate is changed. It could be broken down or combined with another molecule to make something new. It will break or build chemical bonds. When done, you will have the enzyme/products complex.
4. The enzyme releases the product. When the enzyme lets go, it returns to its original shape. It is then ready to work on another molecule of substrate.
Can You Control Them?
Good question! We know what you're thinking: "What if enzymes just kept going and converted every molecule in the world? They would never stop. They would become monsters!" Don’t worry. There are many factors that can regulate enzyme activity, including temperature, activators, pH levels, and inhibitors. We’ll look at enzyme control in the next section.
Controlling Enzymes
Enzymes sometimes need to be controlled. An organism can create its own molecules to slow down and stop the activity of enzymes and proteins. At other times, enzymes can by controlled by poisons and contaminants, such as herbicides. So, what affects enzyme activity?
Temperature: That's a good one.Proteins change shape as temperatures change. Because so much of an enzyme's activity is based on its shape, temperature changes can mess up the process and the enzyme won't work. High enough temperatures will cause the enzyme to denature and have its structure start to break up.
Activators: Sometimes you need an enzyme to work faster. Your body can then create activators. At other times, you might eat something that plays the role of an activator. Activators make enzymes work harder and faster. If you're running in a race and you need more energy, get those enzymes to work! Hormones can trigger responses that activate enzymes.
pH Levels: The acidity of the environment changes the shape of proteins in the same way that temperature does. Do you remember thatpH is a measure of acidity? An increased acidity near an enzyme can cause its shape to change. Those polar and nonpolar amino acids start to twist. If there is enough of a change, the protein could unravel and become totally ineffective.
Inhibitors: These are the opposite of activators. Inhibitors either slow down or stop the activity of an enzyme. They often bond to the protein, changing the overall shape of the enzyme. Remember, when the shape changes, the enzyme will not work the same way. A nasty example of an inhibitor is snake venom or maybe nerve gas from World War I.