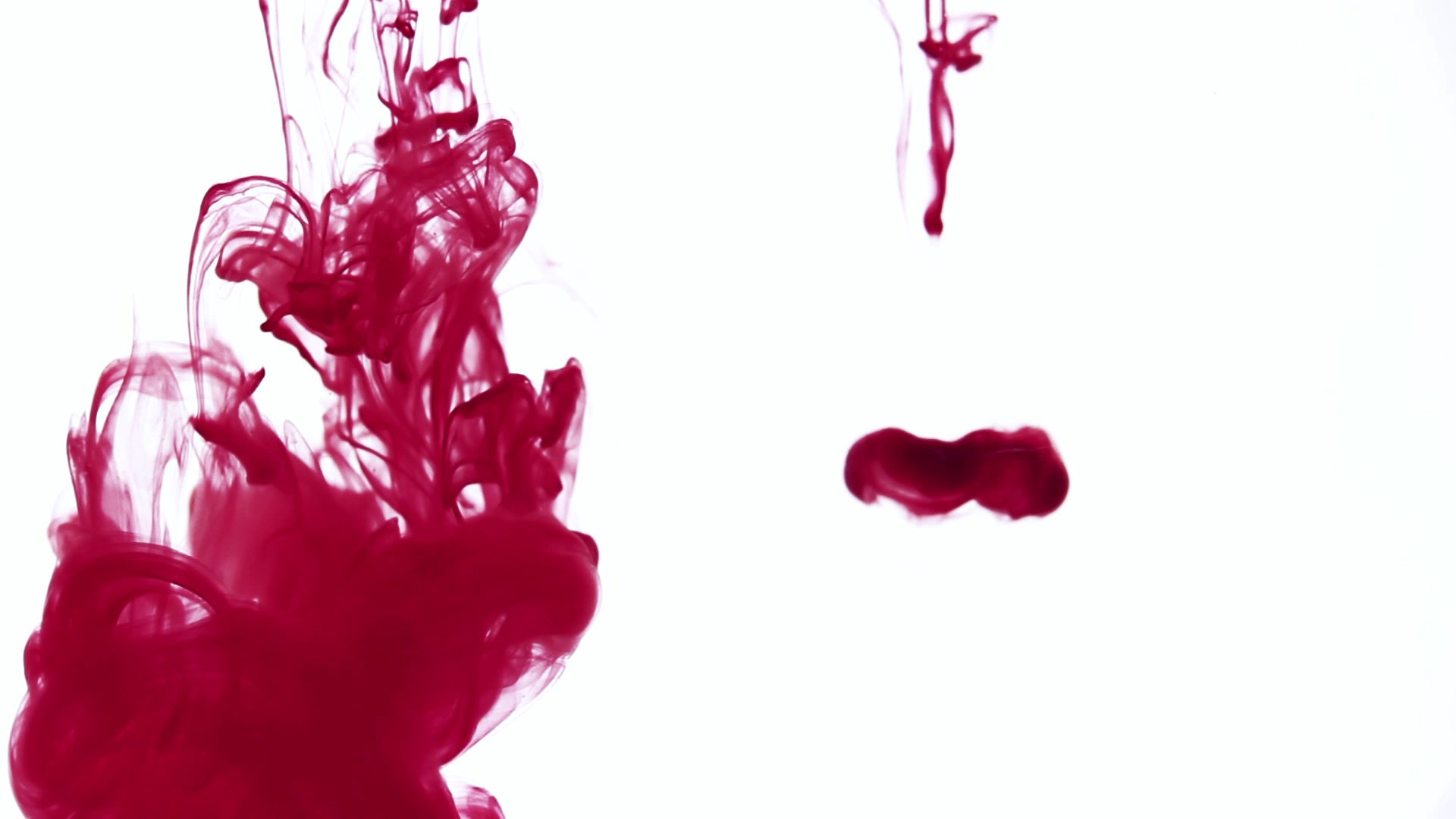
Kissimmee Homeschooling
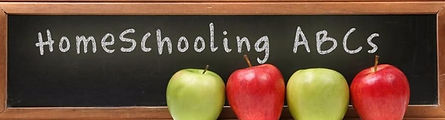

Energy
Enegry Introduction
Energy is one of the most fundamental parts of our universe. We use energy to do work. Energy lights our cities. Energy powers our vehicles, trains, planes and rockets. Energy warms our homes, cooks our food, plays our music, gives us pictures on television. Energy powers machinery in factories and tractors on a farm.
Energy from the sun gives us light during the day. It dries our clothes when they're hanging outside on a clothes line. It helps plants grow. Energy stored in plants is eaten by animals, giving them energy. And predator animals eat their prey, which gives the predator animal energy.
Everything we do is connected to energy in one form or another.
Energy is defined as: "the ability to do work."
What is Energy? - Study Physics
In this video we learn that enery is neither created nor destoryed, but merely is converted. Consider your human particles made from atoms and the energy they produce as they move about in your system. This energy is used while you are living, but what happens to this energy when you are no longer alive? Scientist debate as to what actually occurs after death, but we can understand that although the physical body may be transformed to dust, the spiritual being also made of energy should also go through some form of transformation. This concept is still being widely debated, however Christian scientist propose that the Spiritual man actually is not absorbed back into the Universe but remains intact and returns to the Creator. They also propose that while the body in this current time decays that there will be one day when the Creator of the Universe will call the particles from out of the Universe that were the energy source in your body to reassemble into your physical state. While these concepts seem like pseudoscience (A theory, methodology, or practice that is considered to be without scientific foundation), scienctists now theorize that teleportation and reassembling atoms is possible through the process of entangled quantum physics. While humans do not have the power to reassemble dispursed particles into exact order yet, it is not unreasonable to think that an intelligent Creator would find that impossilbe. This type of science is no longer science fiction and yet many still find it unbelievable to accept the idea that God can easily accomplish what to us seemed at one time unthinkable.
In todays science, Scientist are already attempting some form of this act but the work they are conducting with teleportation systems. Right out of the science fiction movies Star Trek, where people are beemed up from planets to a star ship where their partictles are reassembled. Teleportation, or Teletransportation, is the theoretical transfer of matter or energy from one point to another without traversing the physical space between them. It is a common subject of science fiction literature, film, and television. The use of the term teleport, to describe the hypothetical movement of a person or animal to distant places by means of a wire, has been documented as early as 1878. In a new paper, published in Nature Photonics, scientists reviewed the theoretical ideas around quantum teleportation focusing on the main experimental approaches and their associated advantages and disadvantages. The user can teleport, or transfer matter (beings/objects, including themselves) or energy from one point to another without traversing the physical space between them. This can be achieved by various means, including causing the atoms/molecules to travel at light-speed, warping the space, or use quantum superposition, in which the user teleports by replacing and restocking energy in a spatial behavior.
Lawrence Krauss is an American theoretical physicist and cosmologist who is Foundation Professor of the School of Earth and Space Exploration at Arizona State University and director of its Origins Project. Krauss writes that in order to "dematerialize" something in order to achieve matter teleportation, the binding energy of the atoms and probably that of all its nuclei would have to be overcome. He notes that the binding energy of electrons around nuclei is minuscule relative to binding energy that hold nuclei together. He notes that "if we were to heat up the nuclei to about 1000 billion degrees (about a million times hotter than the temperature at the core of the Sun), then not only would the quarks inside lose their binding energies but at around this temperature matter will suddenly lose almost all of its mass. Matter will turn into radiation—or, in the language of our transporter, matter will dematerialize. In energy units, this implies providing about 10 percent of the rest mass of protons and neutrons in the form of heat. To heat up a sample the size of a human being to this level would require therefore, about 10 percent of the energy needed to annihilate the material—or the energy equivalent of a hundred 1-megaton hydrogen bombs."
Teleportation and Holograms
When we eat, our bodies transform the energy stored in the food into energy to do work. When we run or walk, we "burn" food energy in our bodies. When we think or read or write, we are also doing work. Many times it's really hard work!
Cars, planes, light bulbs, boats and machinery also transform energy into work.
Work means moving something, lifting something, warming something, lighting something. All these are a few of the various types of work.
There are many sources of energy. In The Energy Story, we will look at the energy that makes our world work. Energy is an important part of our daily lives.
The forms of energy we will look at include:
-
Electromagnetic Energy
-
Mechanical Energy
-
Kinetic Energy
-
Potential Energy
-
Elastic Energy
-
Gravitational Energy
-
Electricity
-
Biomass Energy - energy from plants
-
Geothermal Energy
-
Fossil Fuels - Coal, Oil and Natural Gas
-
Hydro Power and Ocean Energy
-
Nuclear Energy
-
Solar Energy
-
Wind Energy
-
Global Warming
Energy - What Is It?
Energy causes things to happen around us. Look out the window. During the day, the sun gives out light and heat energy. At night, street lamps use electrical energy to light our way. When a car drives by, it is being powered by gasoline, a type of stored energy.
The food we eat contains energy. We use that energy to work and play.
"Energy Is the Ability to Do Work."
Energy can be found in a number of different forms. It can be chemical energy, electrical energy, heat (thermal energy), light (radiant energy), mechanical energy, and nuclear energy.
What is a Joule?
The term "joule" is named after an English scientist James Prescott Joule who lived from 1818 to 1889. He discovered that heat is a type of energy. One joule is the amount of energy needed to lift something weighing one pound to a height of nine inches. So, if you lifted a five-pound sack of sugar from the floor to the top of a counter (27 inches), you would use about 15 joules of energy. Around the world, scientists measure energy in joules rather than Btus. It's much like people around the world using the metric system of meters and kilograms, instead of the English system of feet and pounds.
Like in the metric system, you can have kilojoules — "kilo" means 1,000.
1,000 joules = 1 kilojoule = 1 Btu
A piece of buttered toast contains about 315 kilojoules (315,000 joules) of energy. With that energy you could:
-
Jog for 6 minutes
-
Bicycle for 10 minutes
-
Walk briskly for 15 minutes
-
Sleep for 1-1/2 hours
-
Run a car for 7 seconds at 80 kilometers per hour (about 50 miles per hour)
-
Light a 60-watt light bulb for 1-1/2 hours
-
Or lift that sack of sugar from the floor to the counter 21,000 times!
Changing Energy
Energy can be transformed into another sort of energy. Energy cannot be created nort can it be destroyed. Energy has always existed in one form or another.
Here are some changes in energy from one form to another.
Stored energy in a flashlight's batteries becomes light energy when the flashlight is turned on.
Food is stored energy. It is stored as a chemical with potential energy. When your body uses that stored energy to do work, it becomes kinetic energy.
If you overeat, the energy in food is not "burned" but is stored as potential energy in fat cells.
When you talk on the phone, your voice is transformed into electrical energy, which passes over wires (or is transmitted through the air). The phone on the other end changes the electrical energy into sound energy through the speaker.
A car uses stored chemical energy in gasoline to move. The engine changes the chemical energy into heat and kinetic energy to power the car.
A toaster changes electrical energy into heat and light energy. (If you look into the toaster, you'll see the glowing wires.)
A television changes electrical energy into light and sound energy.
Food Energy
Energy changes form at each step in the food chain. Take an ear of corn as an example.
Sunlight is taken in by the leaves on the corn stalk and transformed through photosynthesis. The plant takes in sunlight and combines it with carbon dioxide from the air and water and minerals from the ground.
The plant grows tall and creates the ears of corn - its seeds. The energy of the sunlight is stored in the leaves and inside the corn kernels. The corn kernels are full of energy stored as sugars and starch. The corn is harvested and is fed to chickens and other animals. The chickens use the stored energy in the corn on the cob to grow and to move. Some energy is stored in the animal in its muscle tissue (protein) and in the fat.
The chicken reaches maturity, a farmer slaughters it and prepares it to be sold. It's transported to the grocery store. Your parents buy the chicken at the supermarket, bring it home and cook it (using energy).
You then eat the chicken's meat and fat and convert that stored energy into energy in your own body. Maybe you ate the chicken at a picnic. Then you went and played baseball. You're using the energy from that chicken to swing the bat, run the bases and throw the ball.
As your body uses the energy from the chicken, you breathe in oxygen and exhale carbon dioxide. That carbon dioxide is then used by other plants to grow.
So, it's a big circle!
Heat Energy
Heat is a form of energy. We use it for a lot of things, like warming our homes and cooking our food.
Heat energy moves in three ways:
1. Conduction
2. Convection
3. Radiation
Conduction occurs when energy is passed directly from one item to another. If you stirred a pan of soup on the stove with a metal spoon, the spoon will heat up. The heat is being conducted from the hot area of the soup to the colder area of spoon.
Metals are excellent conductors of heat energy. Wood or plastics are not. These "bad" conductors are called insulators. That's why a pan is usually made of metal while the handle is made of a strong plastic.
Convection is the movement of gases or liquids from a cooler spot to a warmer spot. If a soup pan is made of glass, we could see the movement of convection currents in the pan. The warmer soup moves up from the heated area at the bottom of the pan to the top where it is cooler. The cooler soup then moves to take the warmer soup's place. The movement is in a circular pattern within the pan.
The wind we feel outside is often the result of convection currents. You can understand this by the winds you feel near an ocean. Warm air is lighter than cold air and so it rises. During the daytime, cool air over water moves to replace the air rising up as the land warms the air over it. During the nighttime, the directions change - the surface of the water is sometimes warmer and the land is cooler.
Radiation is the final form of movement of heat energy. The sun's light and heat cannot reach us by conduction or convection because space is almost completely empty. There is nothing to transfer the energy from the sun to the earth.
The sun's rays travel in straight lines called heat rays. When it moves that way, it is called radiation.
When sunlight hits the earth, its radiation is absorbed or reflected. Darker surfaces absorb more of the radiation and lighter surfaces reflect the radiation. So you would be cooler if you wear light or white clothes in the sum.
Solar Energy
Solar power is energy from the sun that is converted into thermal or electrical energy.
Solar energy is the cleanest and most abundant renewable energy source available, and the U.S. has some of the richest solar resources in the world. Modern technology can harness this energy for a variety of uses, including generating electricity, providing light or a comfortable interior environment, and heating water for domestic, commercial, or industrial use.
The U.S. solar market faces both challenges and opportunities; the industry is working to scale up the production of solar technology, and drive down manufacturing and installation costs.
Solar energy is radiant light and heat from the Sun harnessed using a range of ever-evolving technologies such as solar heating,photovoltaics, solar thermal energy, solar architecture and artificial photosynthesis.
It is an important source of renewable energy and its technologies are broadly characterized as either passive solar or active solardepending on the way they capture and distribute solar energy or convert it into solar power. Active solar techniques include the use of photovoltaic systems, concentrated solar power and solar water heating to harness the energy. Passive solar techniques include orienting a building to the Sun, selecting materials with favorable thermal mass or light dispersing properties, and designing spaces that naturally circulate air.
The large magnitude of solar energy available makes it a highly appealing source of electricity.
The Earth receives 174,000 terawatts (TW) of incoming solar radiation (insolation) at the upper atmosphere. Approximately 30% is reflected back to space while the rest is absorbed by clouds, oceans and land masses. The spectrum of solar light at the Earth's surface is mostly spread across the visible and near-infrared ranges with a small part in the near-ultraviolet. Most people around the world live in areas with insolation levels of 150 to 300 watt per square meter or 3.5 to 7.0 kWh/m2 per day.
Solar radiation is absorbed by the Earth's land surface, oceans – which cover about 71% of the globe – and atmosphere. Warm air containing evaporated water from the oceans rises, causing atmospheric circulation or convection. When the air reaches a high altitude, where the temperature is low, water vapor condenses into clouds, which rain onto the Earth's surface, completing the water cycle. Thelatent heat of water condensation amplifies convection, producing atmospheric phenomena such as wind, cyclones and anti-cyclones. Sunlight absorbed by the oceans and land masses keeps the surface at an average temperature of 14 °C. By photosynthesis green plants convert solar energy into chemically stored energy, which produces food, wood and the biomass from which fossil fuels are derived.
The total solar energy absorbed by Earth's atmosphere, oceans and land masses is approximately 3,850,000 exajoules (EJ) per year. In 2002, this was more energy in one hour than the world used in one year. Photosynthesis captures approximately 3,000 EJ per year in biomass. The amount of solar energy reaching the surface of the planet is so vast that in one year it is about twice as much as will ever be obtained from all of the Earth's non-renewable resources of coal, oil, natural gas, and mined uranium combined,
Yearly solar fluxes & human consumption1
Solar 3,850,000
Wind 2,250
Biomass potential~200
Primary energy use 2539
Electricity 2~67
1 Energy given in Exajoule (EJ) = 1018 J = 278 TWh
2 Consumption as of year 2010
The potential solar energy that could be used by humans differs from the amount of solar energy present near the surface of the planet because factors such as geography, time variation, cloud cover, and the land available to humans limits the amount of solar energy that we can acquire. Geography effects solar energy potential because areas that are closer to the equatorhave a greater amount of solar radiation.
Potential and Kinetic Energy
Stored and Moving Energy
Energy makes everything happen and can be divided into two types:
-
Stored energy is called potential energy.
-
Moving energy is called kinetic energy.
Potential energy is the stored energy of position possessed by an object.
Gravitational potential energy is the energy stored in an object as the result of its vertical position or height. The energy is stored as the result of the gravitational attraction of the Earth for the object.
Kinetic energy is the energy of motion. An object that has motion - whether it is vertical or horizontal motion - has kinetic energy.
How do we measure energy?
Energy is measured in many ways. One of the basic measuring blocks is called a Btu. This stands for British thermal unit and was invented by, of course, the English. Is the amount of heat energy it takes to raise the temperature of one pound of water by one degree Fahrenheit, at sea level. One Btu equals about one blue-tip kitchen match. One thousand Btus roughly equals: One average candy bar or 4/5 of a peanut butter and jelly sandwich. It takes about 2,000 Btus to make a pot of coffee. Energy also can be measured in joules. Joules sounds exactly like the word jewels, as in diamonds and emeralds.
A thousand joules is equal to a British thermal unit.
1,000 joules = 1 Btu
So, it would take 2 million joules to make a pot of coffee.
Mathematics of Work
Force and mass information were used to determine the acceleration of an object. A force is a push or pull upon an object resulting from the object's interaction with another object. Whenever there is an interaction between two objects, there is a force upon each of the objects. When the interaction ceases, the two objects no longer experience the force. Mass is a property of a physical body which determines the strength of its mutual gravitational attraction to other bodies, its resistance to being accelerated by a force, and in the theory of relativity gives the mass–energy content of a system. The SI unit of mass is the kilogram (kg). International System of Units is a system of physical units ( SI Units ) based on the meter, kilogram, second, ampere, kelvin, candela, and mole, together with a set of prefixes to indicate multiplication or division by a power of ten. Acceleration information was subsequently used to determine information about the velocity or displacement of an object after a given period of time. In this manner, Newton's laws serve as a useful model for analyzing motion and making predictions about the final state of an object's motion. In this unit, an entirely different model will be used to analyze the motion of objects. Motion will be approached from the perspective of work and energy. The effect that work has upon the energy of an object (or system of objects) will be investigated; the resulting velocity and/or height of the object can then be predicted from energy information. In order to understand this work-energy approach to the analysis of motion, it is important to first have a solid understanding of a few basic terms. Thus, Lesson 1 of this unit will focus on the definitions and meanings of such terms as work, mechanical energy, potential energy, kinetic energy, and power.
When a force acts upon an object to cause a displacement of the object, it is said that work was done upon the object. There are three key ingredients to work - force, displacement, and cause. In order for a force to qualify as having done work on an object, there must be a displacement and the force must cause the displacement. There are several good examples of work that can be observed in everyday life - a horse pulling a plow through the field, a father pushing a grocery cart down the aisle of a grocery store, a freshman lifting a backpack full of books upon her shoulder, a weightlifter lifting a barbell above his head, an Olympian launching the shot-put, etc. In each case described here there is a force exerted upon an object to cause that object to be displaced.
According to Isaac Newton, a force causes only a change in velocity (an acceleration); it does not maintain the velocity.
Newtons Law's of Motion
I. Every object in a state of uniform motion tends to remain in that state of motion unless an external force is applied to it.
II. The relationship between an object's mass m, its acceleration a, and the applied force F is F = ma. Acceleration and force are vectors (as indicated by their symbols being displayed in slant bold font); in this law the direction of the force vector is the same as the direction of the acceleration vector.
III. For every action there is an equal and opposite reaction.
Force = Mass * acceleration
F = m * a
Mechanical Energy
In the process of doing work, the object that is doing the work exchanges energy with the object upon which the work is done. When the work is done upon the object, that object gains energy. The energy acquired by the objects upon which work is done is known as mechanical energy.
Mechanical energy is the energy that is possessed by an object due to its motion or due to its position. Mechanical energy can be either kinetic energy (energy of motion) or potential energy (stored energy of position). Objects have mechanical energy if they are in motion and/or if they are at some position relative to a zero potential energy position (for example, a brick held at a vertical position above the ground or zero height position).
The total amount of mechanical energy is merely the sum of the potential energy and the kinetic energy. This sum is simply referred to as the total mechanical energy (abbreviated TME).
Total mechanical Energy = Potential Energy + Kinetic Energy
TME = PE + KE
As discussed earlier, there are two forms of potential energy discussed in our course - gravitational potential energy and elastic potential energy. Given this fact, the above equation can be rewritten:
Total mechanical Energy = Potential Gravitational Energy (PEgrav) + Potential Elastic Energy (PEspring) + Kinetic Energy
TME = PEgrav + PEspring + KE
A moving car possesses mechanical energy due to its motion (kinetic energy). A moving baseball possesses mechanical energy due to both its high speed (kinetic energy) and its vertical position above the ground (gravitational potential energy). A World Civilization book at rest on the top shelf of a locker possesses mechanical energy due to its vertical position above the ground (gravitational potential energy). A barbell lifted high above a weightlifter's head possesses mechanical energy due to its vertical position above the ground (gravitational potential energy). A drawn bow possesses mechanical energy due to its stretched position (elastic potential energy).
An object that possesses mechanical energy is able to do work. In fact, mechanical energy is often defined as the ability to do work. Any object that possesses mechanical energy - whether it is in the form of potential energyor kinetic energy - is able to do work. That is, its mechanical energy enables that object to apply a force to another object in order to cause it to be displaced.
A common scene in some parts of the countryside is a "wind farm." High-speed winds are used to do work on the blades of a turbine at the so-called wind farm. The mechanical energy of the moving air gives the air particles the ability to apply a force and cause a displacement of the blades. As the blades spin, their energy is subsequently converted into electrical energy (a non-mechanical form of energy) and supplied to homes and industries in order to run electrical appliances. Because the moving wind has mechanical energy (in the form of kinetic energy), it is able to do work on the blades. Once more, mechanical energy is the ability to do work.
Potential Energy
An object can store energy as the result of its position. For example, the heavy ball of a demolition machine is storing energy when it is held at an elevated position. This stored energy of position is referred to as potential energy. Similarly, a drawn bow is able to store energy as the result of its position. When assuming its usual position (i.e., when not drawn), there is no energy stored in the bow. Yet when its position is altered from its usual equilibrium position, the bow is able to store energy by virtue of its position. This stored energy of position is referred to as potential energy. Potential energy is the stored energy of position possessed by an object.
Gravitational Energy
Gravitational potential energy is the energy stored in an object as the result of its vertical position or height. The energy is stored as the result of the gravitational attraction of the Earth for the object. The gravitational potential energy of the massive ball of a demolition machine is dependent on two variables - the mass of the ball and the height to which it is raised. There is a direct relation between gravitational potential energy and the mass of an object. More massive objects have greater gravitational potential energy. There is also a direct relation between gravitational potential energy and the height of an object.
The higher that an object is elevated, the greater the gravitational potential energy. These relationships are expressed by the following equation:
PEgrav = mass • g • height
PEgrav = m *• g • h
In the above equation, m represents the mass of the object, h represents the height of the object and grepresents the gravitational field strength (9.8 N/kg on Earth) - sometimes referred to as the acceleration of gravity. To determine the gravitational potential energy of an object, a zero height position must first be arbitrarily assigned. Typically, the ground is considered to be a position of zero height. But this is merely an arbitrarily assigned position that most people agree upon. Since many of our labs are done on tabletops, it is often customary to assign the tabletop to be the zero height position. Again this is merely arbitrary. If the tabletop is the zero position, then the potential energy of an object is based upon its height relative to the tabletop. For example, a pendulum bob swinging to and from above the tabletop has a potential energy that can be measured based on its height above the tabletop. By measuring the mass of the bob and the height of the bob above the tabletop, the potential energy of the bob can be determined.
Since the gravitational potential energy of an object is directly proportional to its height above the zero position, a doubling of the height will result in a doubling of the gravitational potential energy. A tripling of the height will result in a tripling of the gravitational potential energy.
Elastic Potential Energy
Elastic potential energy is the energy stored in elastic materials as the result of their stretching or compressing. Elastic potential energy can be stored in rubber bands, bungee chords, trampolines, springs, an arrow drawn into a bow, etc. The amount of elastic potential energy stored in such a device is related to the amount of stretch of the device - the more stretch, the more stored energy.
Springs are a special instance of a device that can store elastic potential energy due to either compression or stretching. A force is required to compress a spring; the more compression there is, the more force that is required to compress it further. For certain springs, the amount of force is directly proportional to the amount of stretch or compression (x); the constant of proportionality is known as the spring constant (k).
Force Elastic Energy = Spring constant (k) * Strech or Compression (x)
Fspring = k • x
Such springs are said to follow Hooke's Law. Hooke's law is a principle of physics that states that the force needed to extend or compress a spring by some distance is proportional to that distance. That is: where is a constant factor characteristic of the spring, its stiffness. The law is named after 17th century British physicist Robert Hooke. He first stated the law in 1660 as a Latin anagram (a word, phrase, or sentence formed from another by rearranging its letters). If a spring is not stretched or compressed, then there is no elastic potential energy stored in it. The spring is said to be at its equilibrium position. The equilibrium position is the position that the spring naturally assumes when there is no force applied to it. In terms of potential energy, the equilibrium position could be called the zero-potential energy position. There is a special equation for springs that relates the amount of elastic potential energy to the amount of stretch (or compression) and the spring constant. The equation is
Potential Energy Spring = 0.5 * Spring Constant (k) * (amount of compression2)
PEspring = 0.5 • k • x2
Where
k = spring constant
x = amount of compression
(relative to equilibrium position)
To summarize, potential energy is the energy that is stored in an object due to its position relative to some zero position. An object possesses gravitational potential energy if it is positioned at a height above (or below) the zero height. An object possesses elastic potential energy if it is at a position on an elastic medium other than the equilibrium position.
Kinetic Energy
There are many forms of kinetic energy - vibrational (the energy due to vibrational motion), rotational (the energy due to rotational motion), and translational (the energy due to motion from one location to another). To keep matters simple, we will focus upon translational kinetic energy. The amount of translational kinetic energy (from here on, the phrase kinetic energy will refer to translational kinetic energy) that an object has depends upon two variables: the mass (m) of the object and the speed (v) of the object. The following equation is used to represent the kinetic energy (KE) of an object.
Kinetic Energy = 0.5 * mass * (velocity2)
KE = 0.5 • m • v2
Where
m = mass of object
v = speed of object
This equation reveals that the kinetic energy of an object is directly proportional to the square of its speed. That means that for a twofold increase in speed, the kinetic energy will increase by a factor of four. For a threefold increase in speed, the kinetic energy will increase by a factor of nine. And for a fourfold increase in speed, the kinetic energy will increase by a factor of sixteen. The kinetic energy is dependent upon the square of the speed. As it is often said, an equation is not merely a recipe for algebraic problem solving, but also a guide to thinking about the relationship between quantities. Kinetic energy is a scalar quantity; it does not have a direction.
Unlike velocity, acceleration, force, andmomentum, the kinetic energy of an object is completely described by magnitude alone. Like work and potential energy, the standard metric unit of measurement for kinetic energy is the Joule. As might be implied by the above equation, 1 Joule is equivalent to 1 kg*(m/s)^2.
1 Joule = 1 kg • m2/s2
Power
The quantity work has to do with a force causing a displacement. Work has nothing to do with the amount of time that this force acts to cause the displacement. Sometimes, the work is done very quickly and other times the work is done rather slowly. For example, a rock climber takes an abnormally long time to elevate her body up a few meters along the side of a cliff. On the other hand, a trail hiker (who selects the easier path up the mountain) might elevate her body a few meters in a short amount of time. The two people might do the same amount of work, yet the hiker does the work in considerably less time than the rock climber. The quantity that has to do with the rate at which a certain amount of work is done is known as the power. The hiker has a greater power rating than the rock climber.
Power is the rate at which work is done. It is the work/time ratio. Mathematically, it is computed using the following equation.
Power = Work / time
or
P = W / t
Force
A Force acts on a mass and causes an acceleration. An acceleration causes a change in velocity. Displacement is the Force acted along the direction of the motion. The formula comuting this equation is:
Work = Force * displacement
W = f * d
The Standard Metric Unit Watt
The standard metric unit of power is the Watt. As is implied by the equation for power, a unit of power is equivalent to a unit of work divided by a unit of time. Thus, a Watt is equivalent to a Joule/second. For historical reasons, the horsepower is occasionally used to describe the power delivered by a machine. One horsepower is equivalent to approximately 750 Watts.
Most machines are designed and built to do work on objects. All machines are typically described by a power rating. The power rating indicates the rate at which that machine can do work upon other objects. Thus, the power of a machine is the work/time ratio for that particular machine. A car engine is an example of a machine that is given a power rating. The power rating relates to how rapidly the car can accelerate the car. Suppose that a 40-horsepower engine could accelerate the car from 0 mi/hr to 60 mi/hr in 16 seconds. If this were the case, then a car with four times the horsepower could do the same amount of work in one-fourth the time. That is, a 160-horsepower engine could accelerate the same car from 0 mi/hr to 60 mi/hr in 4 seconds. The point is that for the same amount of work, power and time are inversely proportional. A relationship between two variables in which the product is a constant is called inversely proportional. When one variable increases the other decreases in proportion so that theproduct is unchanged. The power equation suggests that a more powerful engine can do the same amount of work in less time.
A person is also a machine that has a power rating. Some people are more power-full than others. That is, some people are capable of doing the same amount of work in less time or more work in the same amount of time. A common physics lab involves quickly climbing a flight of stairs and using mass, height and time information to determine a student's personal power. Despite the diagonal motion along the staircase, it is often assumed that the horizontal motion is constant and all the force from the steps is used to elevate the student upward at a constant speed. Thus, the weight of the student is equal to the force that does the work on the student and the height of the staircase is the upward displacement. Suppose that Ben Pumpin iron elevates his 80-kg body up the 2.0-meter stairwell in 1.8 seconds. If this were the case, then we could calculate Ben's power rating. It can be assumed that Ben must apply an 800-Newton downward force upon the stairs to elevate his body. By so doing, the stairs would push upward on Ben's body with just enough force to lift his body up the stairs. It can also be assumed that the angle between the force of the stairs on Ben and Ben's displacement is 0 degrees. With these two approximations, Ben's power rating could be determined as shown below.
Ben's power rating is 871 Watts.
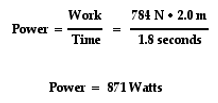
The expression for power is work/time. And since the expression for work is force*displacement, the expression for power can be rewritten as (force*displacement)/time. Since the expression for velocity is displacement/time, the expression for power can be rewritten once more as force*velocity. This new equation for power reveals that a powerful machine is both strong (big force) and fast (big velocity). A powerful car engine is strong and fast. A machine that is strong enough to apply a big force to cause a displacement in a small mount of time (i.e., a big velocity) is a powerful machine. This is shown below.
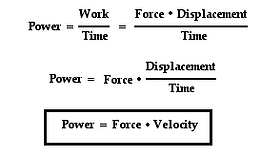
Electrical Energy
Energy is the ability to do work, where work is done when a force moves an object. We need and we use energy every day, and energy is available in all different forms. Electrical energy is energy that's stored in charged particles within an electric field. Electric fields are simply areas surrounding a charged particle. In other words, charged particles create electric fields that exert force on other charged particles within the field. The electric field applies the force to the charged particle, causing it to move - in other words, do work.
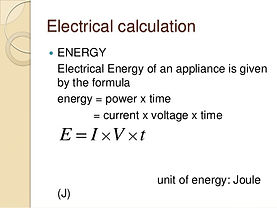
Voltage
Voltage, electric potential difference, electric pressure or electric tension (denoted ∆V or ∆U) is the difference in electric potential energy between two points per unit electric charge. The voltage between two points is equal to the work done per unit of charge against a static electric field to move the charge between two points and is measured in units of volts (a joule per coulomb).
Voltage can be caused by static electric fields, by electric current through a magnetic field, by time-varying magnetic fields, or some combination of these three. A voltmeter can be used to measure the voltage (or potential difference) between two points in a system; often a common reference potential such as the ground of the system is used as one of the points. A voltage may represent either a source of energy (electromotive force), or lost, used, or stored energy (potential drop).
The coulomb (symbolized C) is the standard unit of electric charge in the International System of Units (SI). It is a dimensionless quantity, sharing this aspect with the mole.
A quantity of 1 C is equal to approximately 6.24 x 1018, or 6.24 quintillion.
The mole is a unit of measurement for amount of substance. It is defined as the amount of any chemical substance that contains as many elementary entities, e.g., atoms, molecules, ions, or electrons. The mole may also be used to express the number of atoms, ions, or other elementary entities in a given sample of any substance. The concentration of a solution is commonly expressed by its molarity, defined as the number of moles of the dissolved substance per litre of solution. The mole is widely used in chemistry as a convenient way to express amounts of reactants and products of chemical reactions.
For example, the chemical equation2 H2 + O2 → 2 H2O implies that 2 mol of dihydrogen (H2) and 1 mol of dioxygen (O2) react to form 2 mol of water (H2O).
What are Electric Fields?
Electrical energy is the energy newly derived from electric potential energy. When loosely used to describe energy absorbed or delivered by an electrical circuit (for example, one provided by an electric power utility) "electrical energy" talks about energy which has been converted from electrical potential energy. This energy is supplied by the combination of electric current and electrical potential that is delivered by the circuit. At the point that this electrical potential energy has been converted to another type of energy, it ceases to be electrical potential energy. Thus, all electrical energy is potential energy before it is delivered to the end-use. Once converted from potential energy, electrical energy can always be called another type of energy (heat, light, motion, etc.).
Electric fields point in the direction that positive particles would move within them. Electric fields are like gravitational fields in that both fields are areas surrounding an object that are influenced by the object. A gravitational field surrounds the earth, providing a force that pulls us down. Likewise, electric fields surround charged sources and exert a force on other charged particles that are within the field. Have you ever heard the expression 'opposites attract'? This certainly applies to electric fields. Positive objects create electric fields that repel other positive objects; therefore, the arrows are pointing away from the positive source. Negative sources create electric fields that attract positive objects; therefore, the arrows you see are directed towards the negative source. It's very important to remember the direction of the electric field always points in the direction that a positive particle would move within that field.
Electrical Energy is Potential Energy
Electrical energy is potential energy, which is energy stored in an object due to the object's position. Well, in terms of electrical energy, the object is the charged particle, and the position is the location of that charged particle within the electric field. The charged particle will have the potential to move, or do work, due to the force of the electric field.
Marine Energy
Marine energy or marine power (also sometimes referred to as ocean energy, ocean power, or marine and hydrokinetic energy) refers to the energy carried by ocean waves, tides, salinity, and ocean temperature differences. The movement of water in the world’s oceans creates a vast store of kinetic energy, or energy in motion. This energy can be harnessed to generate electricity to power homes, transport and industries.
The term marine energy encompasses both wave power – power from surface waves, and tidal power – obtained from the kinetic energy of large bodies of moving water. Offshore wind power is not a form of marine energy, as wind power is derived from the wind, even if the wind turbines are placed over water. The oceans have a tremendous amount of energy and are close to many if not most concentrated populations. Ocean energy has the potential of providing a substantial amount of new renewable energy around the world.
Oceans cover more than 70% of Earth's surface, making them the world's largest solar collectors. The sun's heat warms the surface water a lot more than the deep ocean water, and this temperature difference creates thermal energy. Just a small portion of the heat trapped in the ocean could power the world.
Ocean thermal energy is used for many applications, including electricity generation. There are three types of electricity conversion systems: closed-cycle, open-cycle, and hybrid. Closed-cycle systems use the ocean's warm surface water to vaporize a working fluid, which has a low-boiling point, such as ammonia. The vapor expands and turns a turbine. The turbine then activates a generator to produce electricity. Open-cycle systems actually boil the seawater by operating at low pressures. This produces steam that passes through a turbine/generator. And hybrid systems combine both closed-cycle and open-cycle systems.
Ocean mechanical energy is quite different from ocean thermal energy. Even though the sun affects all ocean activity, tides are driven primarily by the gravitational pull of the moon, and waves are driven primarily by the winds. As a result, tides and waves are intermittent sources of energy, while ocean thermal energy is fairly constant. Also, unlike thermal energy, the electricity conversion of both tidal and wave energy usually involves mechanical devices.
A barrage (dam) is typically used to convert tidal energy into electricity by forcing the water through turbines, activating a generator. For wave energy conversion, there are three basic systems: channel systems that funnel the waves into reservoirs; float systems that drive hydraulic pumps; and oscillating water column systems that use the waves to compress air within a container. The mechanical power created from these systems either directly activates a generator or transfers to a working fluid, water, or air, which then drives a turbine/generator.
Geothermal Energy
Geothermal energy is the heat from the Earth. It's clean and sustainable. Resources ofgeothermal energy range from the shallow ground to hot water and hot rock found a few miles beneath the Earth's surface, and down even deeper to the extremely high temperatures of molten rock called magma.
Almost everywhere, the shallow ground or upper 10 feet of the Earth's surface maintains a nearly constant temperature between 50° and 60°F (10° and 16°C). Geothermal heat pumps can tap into this resource to heat and cool buildings. A geothermal heat pump system consists of a heat pump, an air delivery system (ductwork), and a heat exchanger-a system of pipes buried in the shallow ground near the building. In the winter, the heat pump removes heat from the heat exchanger and pumps it into the indoor air delivery system. In the summer, the process is reversed, and the heat pump moves heat from the indoor air into the heat exchanger. The heat removed from the indoor air during the summer can also be used to provide a free source of hot water.
In the United States, most geothermal reservoirs of hot water are located in the western states, Alaska, and Hawaii. Wells can be drilled into underground reservoirs for the generation of electricity. Some geothermal power plants use the steam from a reservoir to power a turbine/generator, while others use the hot water to boil a working fluid that vaporizes and then turns a turbine. Hot water near the surface of Earth can be used directly for heat. Direct-use applications include heating buildings, growing plants in greenhouses, drying crops, heating water at fish farms, and several industrial processes such as pasteurizing milk.
Hot dry rock resources occur at depths of 3 to 5 miles everywhere beneath the Earth's surface and at lesser depths in certain areas. Access to these resources involves injecting cold water down one well, circulating it through hot fractured rock, and drawing off the heated water from another well. Currently, there are no commercial applications of this technology. Existing technology also does not yet allow recovery of heat directly from magma, the very deep and most powerful resource of geothermal energy.
Wind Energy
A wind turbine is a device that converts kinetic energy from the wind into electricalpower. The term appears to have migrated from parallel hydroelectric technology (rotary propeller). The technical description for this type of machine is an aerofoil-powered generator.
We have been harnessing the wind's energy for hundreds of years. From old Holland to farms in the United States, windmills have been used for pumping water or grinding grain. Today, the windmill's modern equivalent - a wind turbine - can use the wind's energy to generate electricity.
Wind turbines, like windmills, are mounted on a tower to capture the most energy. At 100 feet (30 meters) or more aboveground, they can take advantage of the faster and less turbulent wind. Turbines catch the wind's energy with their propeller-like blades. Usually, two or three blades are mounted on a shaft to form a rotor.
A blade acts much like an airplane wing. When the wind blows, a pocket of low-pressure air forms on the downwind side of the blade. The low-pressure air pocket then pulls the blade toward it, causing the rotor to turn. This is called lift. The force of the lift is actually much stronger than the wind's force against the front side of the blade, which is called drag. The combination of lift and drag causes the rotor to spin like a propeller, and the turning shaft spins a generator to make electricity.
Wind turbines can be used as stand-alone applications, or they can be connected to a utility power grid or even combined with a photovoltaic (solar cell) system. For utility-scale sources of wind energy, a large number of wind turbines are usually built close together to form awind plant. Several electricity providers today use wind plants to supply power to their customers.
Stand-alone wind turbines are typically used for water pumping or communications. However, homeowners, farmers, and ranchers in windy areas can also use wind turbines as a way to cut their electric bills.
Small wind systems also have potential as distributed energy resources. Distributed energy resources refer to a variety of small, modular power-generating technologies that can be combined to improve the operation of the electricity delivery system.
Hydropower Energy
Flowing water creates energy that can be captured and turned into electricity. This is called hydroelectric power or hydropower. The most common type of hydroelectric power plant uses a dam on a river to store water in a reservoir.
Water released from the reservoir flows through a turbine, spinning it, which in turn activates a generator to produce electricity. But hydroelectric power doesn't necessarily require a large dam. Some hydroelectric power plants just use a small canal to channel the river water through a turbine.
Another type of hydroelectric power plant - called a pumped storage plant - can even store power. The power is sent from a power grid into the electric generators. The generators then spin the turbines backward, which causes the turbines to pump water from a river or lower reservoir to an upper reservoir, where the power is stored. To use the power, the water is released from the upper reservoir back down into the river or lower reservoir. This spins the turbines forward, activating the generators to produce electricity.
A small or micro-hydroelectric power system can produce enough electricity for a home, farm, or ranch.
Bioenergy
We have used biomass energy or bioenergy - the energy from organic matter - for thousands of years, ever since people started burning wood to cook food or to keep warm. Today, wood is still our largest biomass energy resource. But many other sources of biomass can now be used, including plants, residues from agriculture or forestry, and the organic component of municipal and industrial wastes. Even the fumes from landfills can be used as a biomass energy source.
The use of biomass energy has the potential to greatly reduce our greenhouse gas emissions. Biomass generates about the same amount of carbon dioxide as fossil fuels, but every time a new plant grows, carbon dioxide is actually removed from the atmosphere. The net emission of carbon dioxide will be zero as long as plants continue to be replenished for biomass energy purposes. These energy crops, such as fast-growing trees and grasses, are called biomass feedstocks. The use of biomass feedstocks can also help increase profits for the agricultural industry.
Corn can be harvested to produce ethanol. Unlike other renewable energy sources, biomass can be converted directly into liquid fuels - biofuels - for our transportation needs (cars, trucks, buses, airplanes, and trains). The two most common types of biofuels areethanol and biodiesel. Ethanol is an alcohol, the same found in beer and wine. It is made by fermenting any biomass high in carbohydrates (starches, sugars, or celluloses) through a process similar to brewing beer. Ethanol is mostly used as a fuel additive to cut down a vehicle's carbon monoxide and other smog-causing emissions. But flexible-fuel vehicles, which run on mixtures of gasoline and up to 85% ethanol, are now available.
Biodiesel is made by combining alcohol (usually methanol) with vegetable oil, animal fat, or recycled cooking greases. It can be used as an additive to reduce vehicle emissions (typically 20%) or in its pure form as a renewable alternative fuel for diesel engines. Other biofuels include methanol and reformulated gasoline components. Methanol, commonly called wood alcohol, is currently produced from natural gas, but could also be produced from biomass. There are a number of ways to convert biomass to methanol, but the most likely approach is gasification. Gasification involves vaporizing the biomass at high temperatures, then removing impurities from the hot gas and passing it through a catalyst, which converts it into methanol.
Most reformulated gasoline components produced from biomass are pollution-reducing fuel additives, such as methyl tertiary butyl ether (MTBE) and ethyl tertiary butyl ether (ETBE).
The decay of biomass produces a gas - methane - that can be used as an energy source. In landfills, wells can be drilled to release the methane from the decaying organic matter. Then pipes from each well carry the gas to a central point where it is filtered and cleaned before burning. Methane also can be produced from biomass through a process called anaerobic digestion. Anaerobic digestion involves using bacteria to decompose organic matter in the absence of oxygen.
Methane can be used as an energy source in many ways. Most facilities burn it in a boiler to produce steam for electricity generation or for industrial processes. Two new ways include the use of microturbines and fuel cells. Microturbines have outputs of 25 to 500 kilowatts. About the size of a refrigerator, they can be used where there are space limitations for power production. Methane can also be used as the "fuel" in a fuel cell. Fuel cells work much like batteries but never need recharging, producing electricity as long as there's fuel.
In addition to gas, liquid fuels can be produced from biomass through a process called pyrolysis. Pyrolysis occurs when biomass is heated in the absence of oxygen. The biomass then turns into a liquid called pyrolysis oil, which can be burned like petroleum to generate electricity. A biopower system that uses pyrolysis oil is being commercialized.
Whatever products we can make from fossil fuels, we can make using biomass. These bioproducts, or biobased products, are not only made from renewable sources, they also often require less energy to produce than petroleum-based products.
Researchers have discovered that the process for making biofuels - releasing the sugars that make up starch and cellulose in plants - also can be used to make antifreeze, plastics, glues, artificial sweeteners, and gel for toothpaste.
Other important building blocks for bioproducts include carbon monoxide and hydrogen. When biomass is heated with a small amount of oxygen present, these two gases are produced in abundance. Scientists call this mixturebiosynthesis gas. Biosynthesis gas can be used to make plastics and acids, which can be used in making photographic films, textiles, and synthetic fabrics.
When biomass is heated in the absence of oxygen, it forms pyrolysis oil. A chemical called phenol can be extracted from pyrolysis oil. Phenol is used to make wood adhesives, molded plastic, and foam insulation.
Fossil Fuel Energy
Fossil fuel is a general term for buried combustible geologic deposits of organic materials, formed from decayed plants and animals that have been converted to crude oil, coal, natural gas, or heavy oils by exposure to heat and pressure in the earth's crust over hundreds of millions of years.
Fossil fuels are non-renewable energy sources that formed during the Carboniferous Period. Fossil fuels are made up of plant and animal matter. When plants and animals died, their bodies decomposed and were buried under layers of earth. There are three forms of fossil fuel: oil, natural gasand coal.
Fossil Fuel Energy - Oil
Oil is a thick, black, gooey liquid also called petroleum. It's found way down in the ground, usually between layers of rock. To get oil out, a well is dug. Digging a well is like putting a straw into a can of pop. The oil is then pumped out of the ground, just like when you suck pop up the straw. Oil is carried in pipelines and large tanker ships. A refinery changes the oil into products like gasoline, jet fuel and diesel fuel. It's also burned in factories and power plants to make electricity. The oil is burned, which produces gases that turn a turbine to create electricity.
Fossil Fuel Energy - Natural Gas
Natural gas is lighter than air. Natural gas is made out of methane, which is a simple chemical compound made up of carbon and hydrogen atoms. This gas is highly flammable, so no farting near it. Natural gas is found near oil in the ground. It's pumped, just like oil, from wells that tap into the source and send it to large pipelines. Because you can't smell or see natural gas, it is mixed with a chemical to give it a stinky smell - like rotten eggs. That way, it's easy to tell if there's a leak.
After the stinky chemical is added, the natural gas is sent through underground pipes which go to your home so you can cook food and heat your house. It's also sent to factories and power plants to make electricity. Natural gas is burned to produce heat, which boils water, creating steam, which passes through a turbine to generate electricity.
Fossil Fuel Energy - Coal
Coal comes in several different forms from hard black rocks (that's the kind you get in your stocking at Christmas) to soft brown dirt. Some forms burn hotter and cleaner than others. Coal is used to create more than half of all the electricity made in the US. In the states, many of the coal beds are near the ground's surface. We get to the coal by mining for it. Most coal is transported by trains to power plants where it's burned to make steam. The steam turns turbines, whichproduce electricity.
Nuclear Energy
Flowing water creates energy that can be captured and turned into electricity. This is called hydroelectric power or hydropower. The most common type of hydroelectric power plant uses a dam on a river to store water in a reservoir.
Water released from the reservoir flows through a turbine, spinning it, which in turn activates a generator to produce electricity. But hydroelectric power doesn't necessarily require a large dam. Some hydroelectric power plants just use a small canal to channel the river water through a turbine.
Another type of hydroelectric power plant - called a pumped storage plant - can even store power. The power is sent from a power grid into the electric generators. The generators then spin the turbines backward, which causes the turbines to pump water from a river or lower reservoir to an upper reservoir, where the power is stored. To use the power, the water is released from the upper reservoir back down into the river or lower reservoir. This spins the turbines forward, activating the generators to produce electricity.
A small or micro-hydroelectric power system can produce enough electricity for a home, farm, or ranch.
Global Warming
Global warming is the term used to describe a gradual increase in the average temperature of the Earth's atmosphere and its oceans, a change that is believed to be permanently changing the Earth's climate. Climate change is the greatest humanitarian crisis of our time, responsible for rising seas, raging storms, searing heat, ferocious fires, severe drought, and punishing floods. It threatens our health, communities, economy, and national security.
Global warming and climate change are terms for the observed century-scale rise in the average temperature of the Earth's climate system and its related effects. Multiple lines of scientific evidence show that the climate system is warming. Although the increase of near-surface atmospheric temperature is the measure of global warming often reported in the popular press, most of the additional energy stored in the climate system since 1970 has gone into ocean warming.
The global average (land and ocean) surface temperature shows a warming of 0.85 [0.65 to 1.06] °C in the period 1880 to 2012, based on multiple independently produced datasets. Earth's average surface temperature rose by 0.74±0.18 °C over the period 1906–2005. The rate of warming almost doubled for the last half of that period (0.13±0.03 °C per decade, versus0.07±0.02 °C per decade).
The average temperature of the lower troposphere has increased between 0.13 and 0.22 °C (0.23 and 0.40 °F) per decade since 1979, according to satellite temperature measurements. Climate proxies show the temperature to have been relatively stable over the one or two thousand years before 1850, with regionally varying fluctuations such as the Medieval Warm Period and the Little Ice Age.
The warming that is evident in the instrumental temperature record is consistent with a wide range of observations, as documented by many independent scientific groups. Examples include sea level rise, widespread melting of snow and land ice, increasedheat content of the oceans, increased humidity, and the earlier timing of spring events, e.g., the flowering of plants. Theprobability that these changes could have occurred by chance is virtually zero.
The greenhouse effect is the process by which absorption and emission of infrared radiation by gases in a planet's atmosphere warm its lower atmosphere and surface. It was proposed by Joseph Fourier in 1824, discovered in 1860 by John Tyndall, was first investigated quantitatively by Svante Arrhenius in 1896, and was developed in the 1930s through 1960s by Guy Stewart Callendar.
On Earth, naturally occurring amounts of greenhouse gases have a mean warming effect of about 33 °C (59 °F). Without the Earth's atmosphere, the Earth's average temperature would be well below the freezing temperature of water. The major greenhouse gases are water vapor, which causes about 36–70% of the greenhouse effect; carbon dioxide (CO2), which causes 9–26%;methane (CH4), which causes 4–9%; and ozone (O3), which causes 3–7%. Clouds also affect the radiation balance through cloud forcings similar to greenhouse gases.
Human activity since the Industrial Revolution has increased the amount of greenhouse gases in the atmosphere, leading to increased radiative forcing from CO2, methane, tropospheric ozone, CFCsand nitrous oxide. According to work published in 2007, the concentrations of CO2 and methane have increased by 36% and 148% respectively since 1750. These levels are much higher than at any time during the last 800,000 years, the period for which reliable data has been extracted from ice cores.